fMRI tracks changes in the brain’s oxygen consumption to identify areas of activity, enabling a personalized approach to treating mental illness and developmental disorders. This technology is driving advances in neuroscience research and could have applications in a variety of areas, including early diagnosis and treatment through real-time monitoring, and enhancing creativity and learning.
The function of the brain has long been a subject of study, ever since humans realized its role as the center of movement and thought. Especially in modern times, as the number of brain-related diseases has increased, it has become necessary to study the brain’s activity in depth, and many analytical devices have been invented to meet this need. We are familiar with CT, MRI, PET, and so on.
While these devices have undoubtedly made great strides, they are not perfect. The brain is composed of billions of neurons and 1000 times more synapses. The total number of neurons, the neuronal unit, is an indication of how huge the brain is, and the fact that the number of synapses exceeds the number of neurons makes it very difficult to understand the diversity of connections between neurons. Therefore, to answer the question, “Which part of the brain is responsible for function X?”, the existing instruments were limited by the fact that they showed simple images and were not able to show specific parts. PET, of course, can answer the above questions, but it is too radioactive to be used multiple times.
For this reason, fMRI was developed as an upgrade to MRI. The fMRI utilizes the fact that when the brain performs a certain function, the oxygen consumption in that part of the brain increases significantly, and by tracking this, it helps us to study the functional organization of the brain. Since it only tracks oxygen consumption, there is no risk of exposure to radiation, unlike other imaging modalities, so it is considered a great advantage for freedom of experimentation.
So, how does fMRI identify increased oxygen consumption? To understand this, we need to understand how oxygen is transported in the body. In the body, oxygen is transported by hemoglobin in red blood cells. Red blood cells are proteins made up of billions of hemoglobin, and since hemoglobin binds up to four oxygen molecules, the amount of oxygen supplied to our body is significant. Hemoglobin is therefore divided into two states: unbound and bound to oxygen molecules, which are called deoxyhemoglobin and oxyhemoglobin, respectively. As mentioned above, where there is increased activity in the brain, the proportion of oxyhemoglobin increases, as the amount of oxygen that needs to be delivered to the area increases. The fMRI can quickly find changes in this ratio of hemoglobin states in vivo over a short period of time, allowing researchers to identify active parts of the brain.
Now, let’s think about what fMRI technology can be used for. The brain is divided into five parts: the cerebrum, cerebellum, midbrain, brainstem, and soft brain, of which the midbrain, brainstem, and soft brain, also known as the brain stem, are most closely related to life support. These parts of the brain have been clinically identified through conventional MRIs, and the behavioral aspects of human beings are driven by electrical signals from the nervous system, which have been confirmed through other electrical signal analysis experiments. However, the cerebrum, which is the only part of the brain that controls mental aspects of human beings, cannot be consistently analyzed and is difficult to study due to its myriad possibilities. This is where fMRI’s ability to identify “specific parts” of the brain became a leap forward in analyzing the brain.
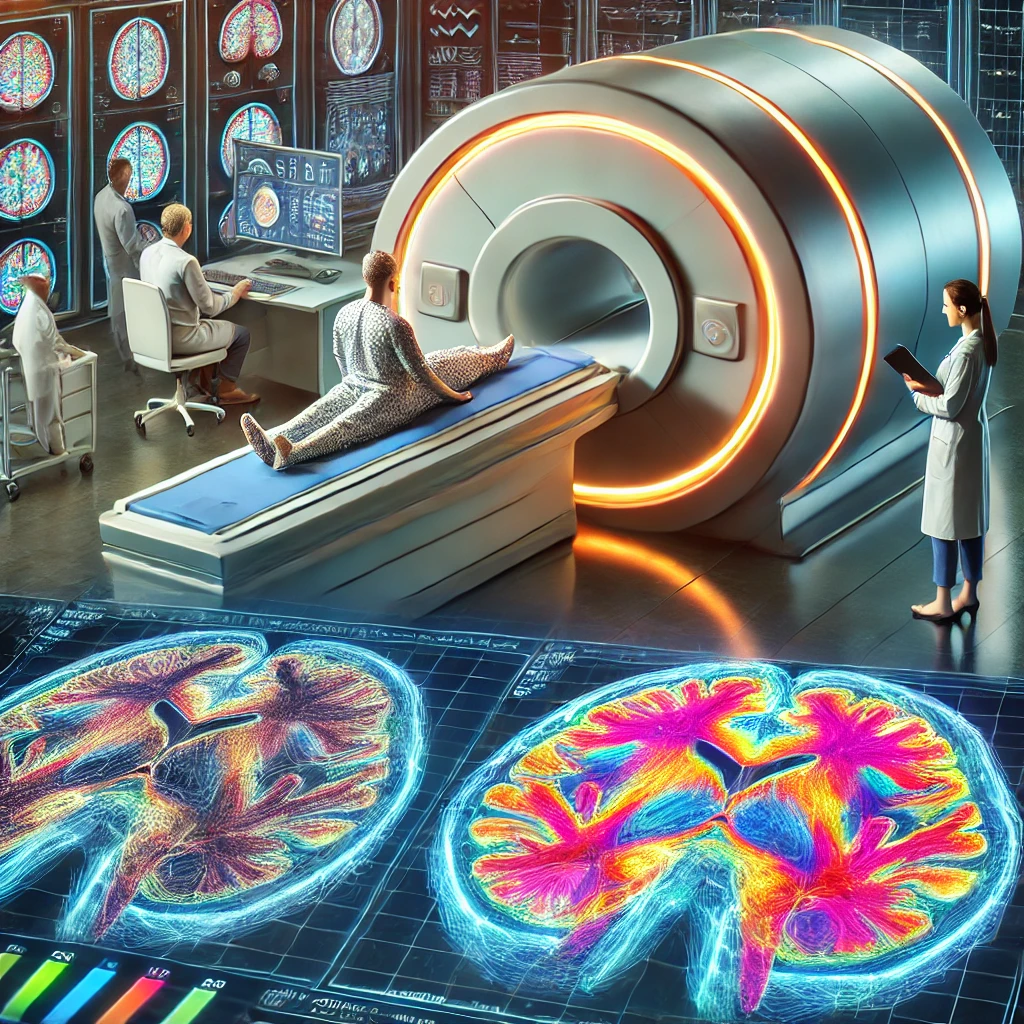
For example, let’s say you want to treat depression. There is no consistent cause of depression. We can infer that depression is caused by a problem in a specific part of the emotional center of the brain, but that’s not accurate. There’s no guarantee that there is a single region responsible for emotional function, and even if there is, there may be localized differences between individuals. In such cases, fMRI can be used to identify the exact part of the brain that is at fault by comparing it to a normal control group, and with the current advances in pharmaceutical technology, targeted dosing is now possible, allowing antidepressants to be administered more effectively and with greater therapeutic benefit.
Broadening the scope of research, fMRI has also been applied to a number of psychiatric disorders that are not specifically labeled. In addition to the mental illnesses we are familiar with, including developmental disorders such as ADHD, there are many cases where the brain can be affected in a very individualized way, and the cause is often unknown. With fMRI, it will be possible to identify these individual brain trouble spots and customize treatment for each disease. Of course, fMRI does not inherently cure the disease itself, but it has eliminated the wrong practice of blindly administering medication without understanding the cause, which has led to many patients suffering adverse effects.
As you can see, fMRI technology has been a revolutionary invention in the advancement of brain science. In recent years, fMRI has become more precise in analyzing the parts of the brain that are used to perform specific tasks, as it can identify changes that occur within microseconds of time. Thanks to these advances, fMRI technology has recently reached the point where it is able to capture detailed processes and brain signals of what areas of the brain are being used in response to audiovisual stimuli in humans, and the recovered audiovisual memories match the original ones to a high degree. The implication of these advances is that fMRI can be used to study the brain with a very high degree of reliability in the future for analyzing the human psyche. In light of this, the development of fMRI that is able to capture more subtle changes with greater freedom of analysis range will become increasingly important, and the potential benefits of such a development are enormous.
Technological advances in fMRI will open up even more possibilities for neuroscience research. For example, real-time monitoring of brain activity could be used to detect early signs of certain diseases, which could play an important role in early treatment and prevention. It could also be used to analyze and enhance human creativity, memory, and learning abilities. This research has many potential applications in education, medicine, psychotherapy, and other fields. Advances in fMRI will eventually become a key tool in improving the quality of human life and building a better future.