Organic Light Emitting Diode (OLED) is a spontaneous light display technology characterized by excellent image quality, low power consumption, and thin, flexible displays. OLEDs use organic materials that are energized by electricity to emit light, where electrons combine with holes in the light-emitting layer to emit light. RGB-OLEDs are composed of red, green, and blue subpixels, and different colors are displayed by adjusting the current in the subpixels. The color of the light is determined by the band gap of the organic material. OLEDs are divided into back-emitting and front-emitting, each with different components, apertures, and luminance.
OLED (Organic Light Emitting Diode) refers to an organic material that emits a specific color of light when energized by electricity in the light emitting layer of an LED. OLEDs have recently gained a lot of attention in display technology due to their excellent image quality, low power consumption, and the ability to enable thin and flexible displays. OLEDs are also self-luminous displays, meaning they don’t require a backlight, allowing for thinner and lighter designs.
The most basic RGB-OLED is made up of three subpixels that emit the three primary colors of light: red, green, and blue. The subpixels are multilayered, with a cathode that injects electrons, a light-emitting layer where electrons and holes meet to produce light, and an anode that injects holes. Each subpixel has a thin-film transistor (TFT) on the positive (+) side that acts as a valve to block or allow current to flow and regulate the amount of current. Turning off all the subpixels produces black and turning on all the subpixels produces white, and by adjusting the amount of current in the subpixels and mixing the amount of light appropriately, various colors of light can be expressed.
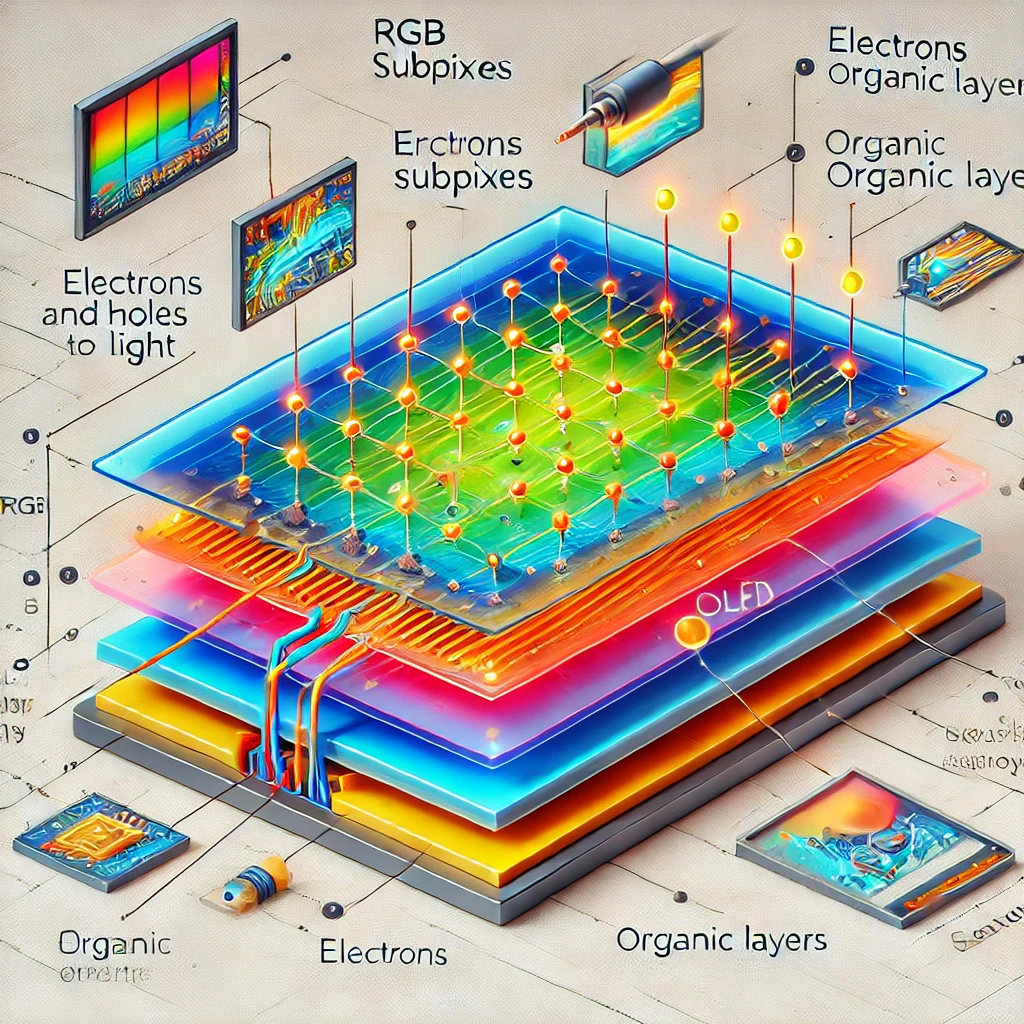
So, how does the light emitting layer produce light? The state in which electrons are stable at the lowest energy is called the ‘ground state’. When a certain amount of energy is applied to the ground state, the electrons move from their original position and become highly energized, which is called the excited state. The electrons in the excited state have the property to stabilize and return to the ground state. At this point, the electrons release energy equal to the difference between the energy of the excited state and the ground state, i.e., the energy that was applied to get from the ground state to the excited state. When a current is applied to the TFT, the excited electrons move toward the anode and the holes toward the cathode until they meet each other in the emission layer. In the emission layer, the electrons combine with the holes and stabilize to form the ground state, where most of the difference in energy between the excited and ground states is converted into light energy.
The color of the light emitted by each subpixel is determined by the ‘band gap’ of the organic material in the emissive layer. The band gap is the difference in energy levels between the highest energy orbital (HOMO) of the electron-filled region and the lowest energy orbital (LUMO) of the electron-filled region. When an electron that is ground in the HOMO is energized above the band gap, the excited electron moves to the LUMO and bonds with a hole. The electron then releases energy and returns to the ground state, emitting light at a wavelength corresponding to the band gap. Because larger band gaps require more energy to emit light, organic materials with larger band gaps have shorter lifetimes than those with smaller band gaps.
OLEDs are divided into back-emitting and front-emitting depending on where the light produced by the emissive layer in the middle is directed. If the light is directed toward the anode, it is called back-emitting, and if it is directed toward the cathode, it is called front-emitting. In the case of backlighting, the cathode must act as an electron injection and reflection layer, so a mixed metal of silver and magnesium is used, which has a low work function and is opaque. The anode, on the other hand, is a compound of indium and tin oxide (ITO), which has the opposite properties. However, when light passes through the TFT on the anode, some of the light is blocked by the TFT and cannot escape, resulting in a decrease in aperture. Aperture is the ratio of the area that can actually emit light to the total area of a unit pixel, and a high aperture means that the amount of light emitted when the same current is applied is high, resulting in high brightness. Because of this, a decrease in aperture leads to a decrease in brightness, which is detrimental to the lifespan of organic materials because they have to light up more brightly to make up for the lost brightness to produce the same image quality.
In order to increase the aperture, front-emitting, where light is directed toward the cathode without a TFT, requires the use of a metal such as gold or platinum for the anode, which has a high work function and can act as a reflective layer, and a highly transparent material for the cathode. However, if ITO is used for the cathode, it has a high work function and cannot easily give up electrons. As a result, we need to use a metal with a low work function and high transparency for the cathode, and to increase the transparency, we need to make the metal thinner. However, if the cathode is thinned below a certain thickness, the surface resistance increases, and as the resistance increases, the voltage generated by each position of the panel varies, which has the side effect of reducing the uniformity of the screen.
A typical way to solve this problem is to use the phenomenon of microresonance. Some of the light generated by the emissive layer escapes through the translucent cathode, but some of it is reflected by the cathode, directed toward the anode, and re-reflected back to the anode, causing the reflected light to interfere with each other, resulting in microresonance. When waves that are in phase with each other, the light is subjected to reinforcing interference, which makes the waves stronger, and when waves that are out of phase with each other, they are subjected to canceling interference, which makes the waves weaker or disappear. This microresonance phenomenon allows the luminance to increase as the intensity of light increases, resulting in a longer lifespan of the OLED because it does not need to be driven with high current to improve the luminance. In addition, only the wavelengths that match the conditions are enhanced while those that do not are canceled out, resulting in a narrower spectrum and thus a higher purity of color.