Nuclear engineering is a broad field of study that is not just limited to the advancement of nuclear energy. It encompasses many areas of research, including nuclear systems engineering, radiation engineering, fusion and plasma research, and contributes to many industries such as healthcare, materials science, environmental remediation, and space exploration.
Many people have a preconceived notion that nuclear engineering is the study of nuclear energy only. This is due to a lack of understanding of nuclear engineering, which encompasses a wide range of research areas that allow us to contribute to a variety of industries and technological advances beyond energy. Our department has three main research areas. The first is nuclear systems engineering, which involves the control and utilization of nuclear energy from nuclear fission. The second area of research is radiation engineering, which deals with and utilizes radiation from nuclear fission. Finally, there is the field of fusion and plasma, which is of interest to many majors. As you can see, nuclear engineering is not just about obtaining energy, but also about studying various applications of radiation and plasma.

Nuclear fission, the central focus of nuclear systems engineering, and nuclear power plants utilize uranium (U), the heaviest naturally occurring element on Earth. An atom consists of a nucleus, made up of neutrons and protons, and electrons orbiting around it. Because there are only protons and neutrons in the nucleus, the protons repel each other. Energy is required to hold the protons and neutrons together and keep them in a stable state, which is called binding energy. Binding energy is stored in the form of mass according to Einstein’s formula. When the nucleus of an atom splits, mass is converted into energy and a huge amount of binding energy is released. Nuclear power plants generate electricity by fissioning uranium into neutrons to obtain binding energy. Nuclear systems engineering studies the core reactor, where fission occurs in nuclear power plants, and how to efficiently utilize the energy gained from fission.
When atomic nuclei split, electromagnetic waves in the form of particles or light are emitted along with energy, which is called radiation. Some types of radiation have high energy and are dangerous, so sometimes they need to be controlled, but sometimes they can be useful in real life, such as X-rays, so engineering to study and utilize radiation is also an important field of study. Radiation engineering studies how to safely manage this radiation and utilize it for a variety of medical and industrial applications. For example, radiation therapy plays an important role in cancer treatment, and radiation imaging is essential for diagnosing disease.
Nuclear Fusion – Primarily addressed in the plasma field, nuclear fusion energy is obtained and utilized by similar principles to nuclear fission. While nuclear fission utilizes uranium and neutrons, nuclear fusion utilizes deuterium and tritium. While most hydrogen atoms have one proton in their nucleus, deuterium and tritium are hydrogen atoms that have one and two additional neutrons attached to their nuclei, respectively. When deuterium and tritium nuclei bump into each other, they turn into helium and neutrons. The binding energy required to stabilize a helium nucleus is less than the binding energy required to stabilize a deuterium nucleus, so the excess binding energy is released in nuclear fusion.
Nuclear fusion has many advantages over fission. While uranium is a limited resource, deuterium and tritium are more abundant than uranium. 0.1% of all hydrogen is deuterium, which can be extracted from water, and tritium can be easily obtained from lithium. Nuclear fusion energy is also more energy dense than nuclear fission energy. In addition, when uranium is fissioned, it splits into two or three elements, which are unstable and emit various forms of radiation before turning into stable elements. This requires multiple methods to safely dispose of the unstable elements, and if not all of them are disposed of, they can be released during a natural disaster such as an earthquake, causing serious damage, such as the Fukushima accident. Nuclear fusion, however, is radiation-safe because it only produces helium and neutrons.
But there’s a catch. In fission, neutrons are not subject to electromagnetic forces, so they have no obstacles to reach the nucleus of a uranium atom. In fusion, however, the nuclei of deuterium and tritium have protons, so when they come close to each other, they repel. In order to overcome this repulsion and fusion can occur, the kinetic energy of deuterium and tritium must be very high, which means that high temperatures are needed to increase the kinetic energy of the atoms, which puts the atoms into a plasma state where the protons, neutrons, and electrons are separated from each other. Because plasma is so hot and easily blown away, it needs to be confined within sophisticated and detailed electric and magnetic fields in order to be harnessed. As a result, nuclear fusion requires the development of technologies that can manipulate and harness plasma.
And while plasma is an important precursor to nuclear fusion, it is so useful in engineering that it has become an important field of research in its own right and has many impacts on our daily lives. For example, plasma is used to make semiconductors, which are precisely shaved down to the nanometer scale, and molecules that make lenses and eyeglasses hard are made into plasma to permeate the surface of the lenses, making them harder and less prone to scratches.
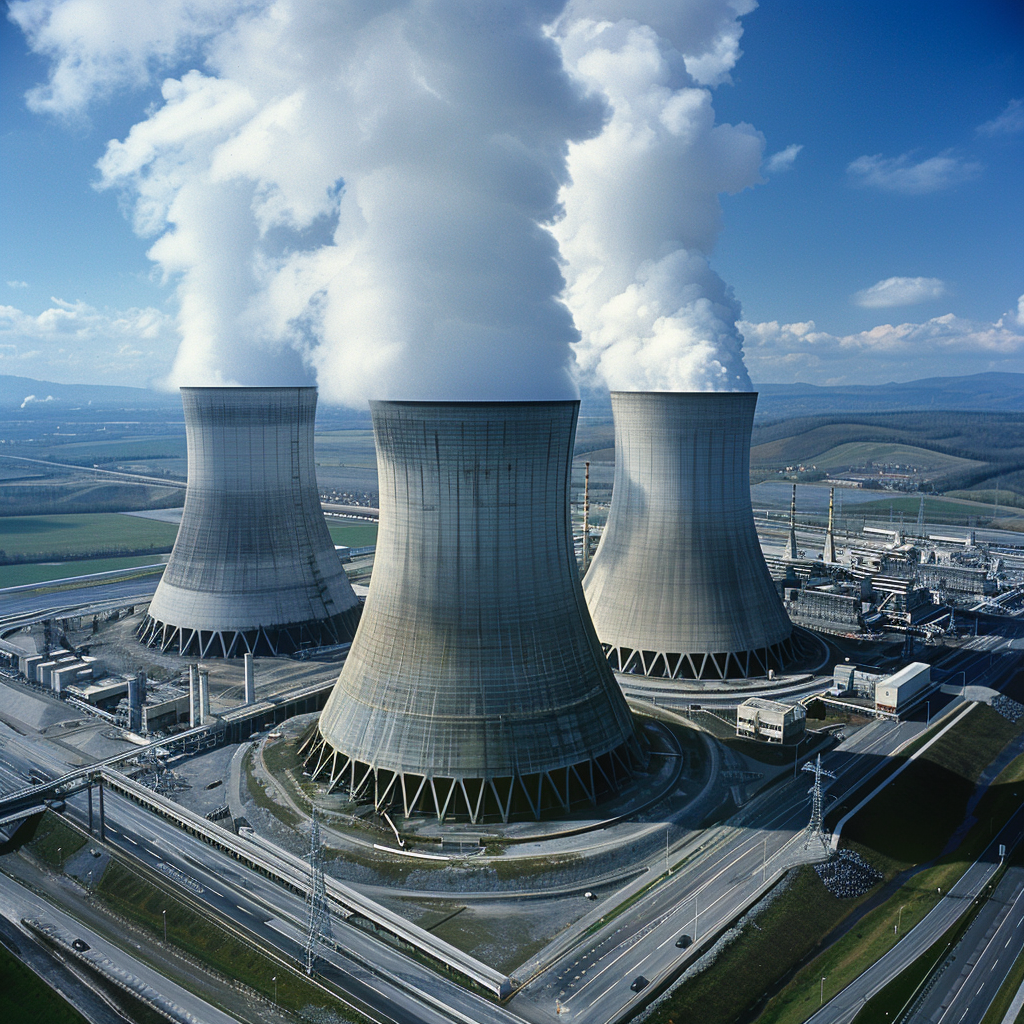
Nuclear engineering has such a wide range of research fields and applications, and will play a huge role in future technological advancements and solving energy problems. This diversity of disciplines is why nuclear engineering can play an important role in many industries and disciplines beyond just energy engineering. Therefore, it is important to broaden your understanding of nuclear engineering and take an interest in its various research fields.